阅读:0
听报道
撰文 | Frank Wilczek
翻译 | 胡风、梁丁当
中文版
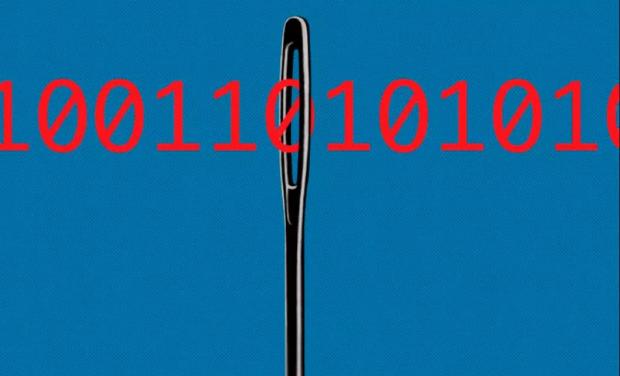
现代计算一直在尝试模仿自然界成功的纠错模式。或许量子效应会提供新的解决方案。
“精确”是一个强大的工具,但它常常很难实现。关于“精确”的主题一直是科学、生命和现代技术的主旋律。而今,在量子计算的前沿,这个旋律再次响起。
在生物界中,复杂机体把它们的基本操作系统——即如何构建细胞并使之运行的指令——存储在长串的DNA分子中。这些基本指令被读取、翻译成相应的化学反应。如果翻译出了错,其后果可能是灾难性的,会产生有缺陷、功能紊乱的蛋白质,甚至致癌。因此,生物界演化出了一套精准的修复与勘误机制,能使错误率维持在十亿分之一左右。在这个校正过程中,有一系列复杂的分子机器监控着翻译的进程并进行纠错。这种分子机器的产生是生物演化过程中最伟大的成就之一。
计算机的许多应用也要求精确。(比如,在银行交易中,准确的密码是非常重要的!)当可靠的微型固态晶体管出现时,现代计算机技术便应运而生了。在晶体管中,电子被储存在一个“容器”里。这个容器的两个不同位置编码了“0”与“1”。如果容器里的电子足够多,那么少数几个电子的位置错误并不会破坏信息的准确性。
但在计算过程中,计算机必须移动这些容器。容器越小,就越容易移动。事实上,计算机运行速度的不断刷新,很大程度上是因为制造单个比特的电子数在不断减少。目前的前沿技术已达到10个电子甚至更少的水平。可与此同时,大量“冗余”电子所带来的抗误差能力也随之消失。要继续维持几乎完美、精确的操作,就需要新的技巧。
基础物理还带来了另一个问题。当接近单电子的层面时,量子力学的影响变得更加突出。对我们来说,这既是挑战,也可能是福音。
量子力学告诉我们,电子的位置不是确定的,而是以一定的几率分布。这种量子性进一步模糊了关键的0与1的区别。但祸兮福所倚,这也带来了潜在的好处,即我们可以尝试利用量子物质的复杂性来达成目标。这就是量子计算的愿景。目前,世界各地都在积极开展相关研究,旨在为量子计算创建可用的平台。
量子计算最大的挑战是如何获得足够高的计算精度。目前人们试图通过两种策略来实现这一目标。
第一种策略是类似于生物的方法,即允许错误的发生,但同时也在努力地纠错。不幸的是,有效的纠错需要大量“冗余”和复杂的纠错机制,因此通过这种途径来实现“精确”会比较痛苦。
另一种策略则是所谓的“拓扑量子计算”。这是一种很前沿的技术,它的前提是制造出所谓的“任意子” (anyon) ,这种粒子可以被看作一团团有记忆的能量。人们希望制造出使用任意子而不是电子的新型晶体管。但直到最近,物理学家才成功地制造出了任意子,而它们的实际应用前景还尚待观察。
我们能否继续应对精确性的挑战?自然界本身让我们坚定了信心——毕竟,大自然已经完美地“制造”了大量可交换的部件(比如电子),并以完美的精确度“计算”出了它们的行为。
英文版
Modern computing has sought to mimic the error-correction success of the natural world, and quantum weirdness may now provide new solutions.
Precision is a powerful tool, but it can be hard to come by. That theme, with variations, is a leitmotif of science, organic life and modern technology. It is sounding again today, at the frontier of quantum computing.
Consider biology. Complex organisms store their essential operating systems—instructions for how to build cells and keep them going—within long DNA molecules. Those basic programs must be read out and translated into chemical events. Errors in translation can be catastrophic, resulting in defective, dysfunctional proteins or even in cancers. So biology has evolved an elaborate machinery of repair and proofreading to keep error rates low—around one per billion operations. A series of complicated molecular machines examine the progress and correct mistakes, in a process aptly called proof-reading. The creation of this machinery is one of evolution’s greatest achievements.
Many applications of computers also need precision. (For instance, in bank transactions it’s important to get passwords and transfers exactly right!) Modern computer technology came into its own when small, reliable solid-state transistors became available. Here, the basic distinction between “0” and “1” gets encoded in two alternative locations for buckets of electrons. When there are many electrons per bucket, errors in the position of one or a few don’t spoil the message.
But in doing computations the computer must move the buckets around. Making those buckets of electrons smaller makes the job of moving them around easier. Indeed, the computer industry’s spectacular record of ever-faster speed is largely the story of lowering the number of electrons used to make a bit; nowadays we’re approaching ten or fewer. Unfortunately, at this frontier the near error-immunity that stems from having many “redundant” electrons is less automatic. To maintain nearly error-free, precise operation, new tricks will be necessary.
Fundamental physics brings in another issue. As you approach the level of single electrons, the effects of quantum mechanics become more prominent. This is both a challenge and, potentially, a blessing.
In quantum mechanics, we learn that electrons do not have definite positions but rather distributions of probability. This further blurs the crucial 0-1 distinction. The potential blessing is the flip side of that coin. We can try to exploit the complexity of matter that quantum theory reveals for useful purposes. This is the vision of so-called quantum computing. Vigorous research efforts aimed at providing useful platforms for quantum computing are in progress around the world.
The great challenge is to reach high precision. People are pursuing two kinds of strategies, broadly parallel to those in biology and classical computing.
The first, quasi-biological approach is to let some errors happen but to work hard to correct them. Unfortunately, good error correction requires redundancy and lots of complex machinery, so this path to precision is painful.
The second, called topological quantum computing, is an avant-garde technology. It is premised on making buckets of energy that have a kind of memory—so-called “anyons.” People hope to construct new kinds of transistors that use anyonics rather than electronics. But only very recently have physicists succeeded in producing anyons at all, and it remains to be seen if they can be put to practical use.
Can we continue to meet the challenge of precision? Nature herself inspires faith, for Nature “manufactures” perfectly interchangeable parts (e.g., electrons) in vast quantities and “calculates” their behavior—with perfect accuracy.
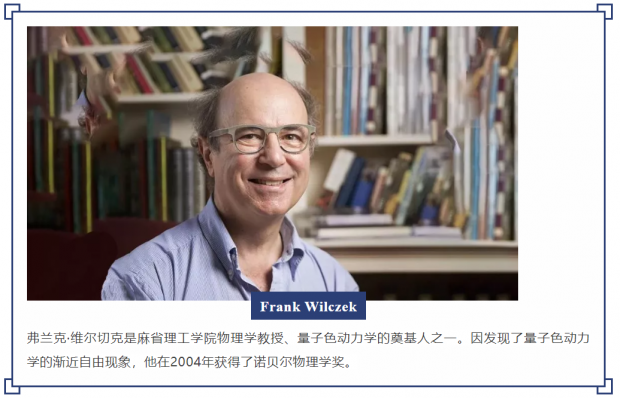
本文经授权转载自微信公众号“蔻享学术”。
话题:
0
推荐
财新博客版权声明:财新博客所发布文章及图片之版权属博主本人及/或相关权利人所有,未经博主及/或相关权利人单独授权,任何网站、平面媒体不得予以转载。财新网对相关媒体的网站信息内容转载授权并不包括财新博客的文章及图片。博客文章均为作者个人观点,不代表财新网的立场和观点。